Speakers
The NIN Summer School features lectures from 12 internationally recognized top-level scientists.
Speakers
The NIN Summer School features lectures from 12 internationally recognized top-level scientists.
- E. J. Chichilnisky
- Tracy Cui
- Karl Deisseroth
- Andreas Horn
- Kristen Kozielski
- Stéphanie Lacour
- Helen Mayberg
- Serge Picaud
- Nick Ramsey
- Andy Schwartz
- Michael Shadlen
- Alan Urban
E.J. Chichilnisky is the John R. Adler Professor of Neurosurgery, and Professor of Ophthalmology, at Stanford University, where he has worked since 2013. Previously, he worked at the Salk Institute for Biological Studies for 15 years. He received his B.A. in Mathematics from Princeton University, and his M.S. in mathematics and Ph.D. in neuroscience from Stanford University. His research has focused on understanding the spatiotemporal patterns of electrical activity in the retina that convey visual information to the brain, and their origins in retinal circuitry, using large-scale multi-electrode recordings. His ongoing work now focuses on using basic science knowledge along with electrical stimulation to develop a novel high-fidelity artificial retina for treating incurable blindness.
Toward a High-fidelity Artificial Retina
Electronic interfaces to the retina represent an exciting development in science, engineering, and medicine – an opportunity to exploit our knowledge of neural circuitry and function to restore or even enhance vision. However, although existing devices demonstrate proof of principle in treating blindness, they produce limited visual function. Some of the reasons for this can be understood based on the precise and specific neural circuitry that mediates visual signaling in the retina. Consideration of this circuitry suggests that future devices may need to operate at single-cell, single-spike resolution in order to subserve naturalistic visual function. I will show large-scale multi-electrode recording and stimulation data from the macaque and human retina indicating that, in some cases, such resolution is possible. I will also discuss cases in which it fails, and propose that we can improve artificial vision in such conditions by incorporating our knowledge of the visual system in bi-directional devices that adapt to the host neural circuitry. Finally, I will introduce the Stanford Artificial Retina Project, aimed at developing a retinal implant that more faithfully reproduces the neural code of the retina, and briefly discuss the implications for scientific investigation and for other neural interfaces of the future.
When: Saturday June 4 @ 10:15 am
Tracy Cui is professor of bioengineering in the Swanson School of Engineering in Pennsylvania. She runs the Neural Tissue/Electrode Interface and Neural Tissue Engineering Lab, where they develop new engineering tools to study and clinically control the interface between tissue and implanted neural devices.
Biomaterials Strategies Towards Seamless Neural Tissue-Device Interface
Neuroelectronic devices placed in the nervous system to record and modulate neuroactivity or monitor neurochemical concentrations present tremendous potentials for understanding neural circuits and treating neurological diseases. Currently, the performance of these devices is sub-optimum due to electrode material limitations and undesired host tissue responses. Quantitative histology and 2-photon imaging have revealed neuronal damage and degeneration, inflammatory gliosis, blood brain barrier leakage and oxidative stress at the site of implants which may compromise the intended recording/stimulation/sensing functions. We use several biomaterial strategies to minimize these responses in order to achieve seamless and stable device-tissue interface. First, conducting polymer-based nanocomposites have been investigated as electrode coatings to facilitate the signal transduction/charge transfer between the ionically conductive tissue and the electronic device. We employ nanotechnology to improve the stability, charge injection and drug delivery capability of the conducting polymers to meet the material challenges. Secondly, materials and devices that mimic the mechanical properties of the neural tissue have been developed and shown to significantly reduce the chronic inflammation. Thirdly bioactive approaches are being developed to modulate the cellular responses for seamless integration. Biomimetic surface modifications have been found to significantly improve neuronal health and inhibit the inflammatory tissue response around the implants. Alternatively, therapeutics that control inflammation, neurodegeneration and oxidative stress can be delivered systemically or locally. These bioactive approaches demonstrated significant effects in improving neural interface performance. The ultimate solution to a seamless device/tissue interface may be a combinatorial approach that takes advantage of multiple biomaterial strategies discussed above and beyond.
When: Saturday, June 4 @ 3:05 pm
Karl Deisseroth is the D.H. Chen Professor of Bioengineering and of Psychiatry and Behavioral Sciences at Stanford University, and Investigator of the Howard Hughes Medical Institute. He received his undergraduate degree from Harvard, his PhD from Stanford, and his MD from Stanford. He also completed postdoctoral training, medical internship, and adult psychiatry residency at Stanford, and he is board-certified by the American Board of Psychiatry and Neurology. He continues as a practicing psychiatrist at Stanford with specialization in affective disorders and autism-spectrum disease, employing medications along with neural stimulation.
Over the last sixteen years, his laboratory created and developed optogenetics, hydrogel-tissue chemistry (beginning with CLARITY), and a broad range of enabling methods. He also has employed his technologies to discover the neural cell types and connections that cause adaptive and maladaptive behaviors, and has disseminated the technologies to thousands of laboratories around the world.
The inner workings of brains and channelrhodopsins
I will discuss the development and application of new optogenetic methods, including molecular tools and brainwide recording approaches (both optical and electrical) in mouse and human; insights into complex behavior that result include a deeper understanding of the mysterious state of dissociation, with both basic-science and clinical implications for the most high-level and integrative roles of the mammalian brain.
I will also explore the remarkable family of light-activated ion channels, or channelrhodopsins, which span three main groups (the cation channels, the anion channels, and the pump-like channels). We have previously described the first high-resolution structures of the first two groups (cation and anion channels), and used these insights to redesign the channels for new kinds of function in optogenetics, but the third family of channelrhodopsins had remained mysterious. For example, ChRmine (the paradigmatic member of this pump-like channelrhodopsin family, for which we reported initial discovery in 2019) exhibits puzzling properties (unusually-large photocurrents, exceptional red-shift in action spectrum, and extreme light-sensitivity) that opened up new opportunities in optogenetics, including the initial definition and control of specific mammalian perceptions at single cell resolution. In this talk I will present our new cryo-electron microscopy structure of ChRmine at 2.0 Å resolution. The structure reveals striking architectural features never seen before in channelrhodopsins including trimeric assembly, a short transmembrane-helix 3 unwound in the middle of the membrane, a prominently-twisting extracellular-loop 1, remarkably-large intracellular cavities and extracellular vestibule, and an unprecedented hydrophilic pore that extends through the center of the trimer, separate from the three individual monomer pores. These structurally distinct features that may enable ChRmine (representing the third of the three major branches of the channelrhodopsin family) to function as an especially potent ion channel; we therefore applied the high-resolution structure to design and create two new proteins (rsChRmine and hsChRmine, for red-shifted and high-speed optogenetic performance, respectively).
Insights into complex behavior that have emerged from Ångstrom-level understanding of these proteins include deeper understanding of the fundamental survival drives of animals at single-cell resolution, and of the highest-level integrative functions of the brain, with both basic-science and clinical implications.
When: Thursday, June 2 @ 4:00 pm
Andreas is a clinician scientist with interest in neuroimaging, methods development, movement disorders and invasive brain stimulation. The goal of his research is to analyze and modulate brain networks to improve clinical treatment – predominantly in the movement disorders spectrum.
Connectomic Deep Brain Stimulation
In recent years, large initiatives around the globe have accumulated data used to calculate average wiring diagrams of the human brain. These connectomes may also be used to investigate clinical populations and have specific value for deep brain stimulation, a procedure in which focal areas in the depth of the brain are being modulated by electrodes invasively implanted into the brains of patients.
These focal stimulations in turn lead to global network effects, often altering activity in distributed whole-brain functional brain networks. Using the human connectome, we are now poised to investigate exactly which networks seem to matter in order to achieve maximal benefits for each patient.
In this talk, I will review methods and results from multiple studies that research labs world wide have applied to study connectomic effects of focal neuromodulation. We will cover results in diseases ranging from the movement disorders spectrum (Parkinson’s Disease, Dystonia, Essential Tremor) to neuropsychiatric (Tourette’s Disease) and psychiatric (Obsessive Compulsive Disorder, Depression) diseases. I will also demonstrate how findings in seemingly different diseases (such as Parkinson’s Disease and Depression) could be transferred to cross-inform one another.
When: Friday, June 3 @ 9:00 am
Kristen Kozielski is an assistant professor of neuroengineering at the Technical University of Munich (TUM) in the Department of Electrical and Computer Engineering. She previously ran the Neuroelectronic Materials Group within the Institute of Functional Interfaces at the Karlsruhe Institute of Technology (KIT). Dr. Kozielski completed her PhD in biomedical engineering at Johns Hopkins University, and her postdoctoral research at the Max Planck Institute for Intelligent Systems. At TUM, her group works with new materials for wireless communication in the brain and nervous system. The goal of this work is to contribute to neurotechnologies that are minimally invasive and can potentially be implanted without surgical intervention. Dr. Kozielski’s work has received funding from several national and international awards, including the National Institutes of Health. She has authored 27 peer-reviewed publications, and her work has been cited over 1300 times.
Magnetoelectric nanomaterials for wireless neuronal modulation
Prof. Kristen Kozielski works with new materials for wireless communication with the brain and nervous system. Her research focuses on understanding and optimizing materials for controlled, electronic signaling to and from the brain. The goal of this work is to contribute to neurotechnologies that are minimally invasive, and possibly implanted with no surgical intervention. Her multidisciplinary group works in materials science, biomaterials, nanotechnology, electrical engineering, and neurobiology.
Kristen completed her PhD in Biomedical Engineering at Johns Hopkins University, and her postdoctoral research at the Max Planck Institute for Intelligent Systems. She moved to the Karlsruhe Institute of Technology in 2019 as a group leader. She has been at the Technical University of Munich (TUM) since 2021, where she is an Assistant Professor of Neuroengineering Materials in the Department of Electrical and Computer Engineering. At TUM, she is also the Program Director of the Elite Master’s Program in Neuroengineering.
When: Saturday, June 4 @ 4:40 pm
Stéphanie P. Lacour is full professor at the School of Engineering at the Ecole Polytechnique Fédérale de Lausanne. She received her PhD in Electrical Engineering from INSA de Lyon, France, and completed postdoctoral research at Princeton University (USA) and the University of Cambridge (UK). She joined EPFL in 2011. She is a co-founding member and current director of EPFL Center for Neuroprosthetics, located at EPFL satellite – Campus Biotech in Geneva.
She is the recipient of the 2006 MIT TR35, the 2011 Zonta award, and she was selected as one of the 2015 WEF Young Global Leaders. She was awarded the ERC Starting Grant (2011), ERC POC Grants (2016 & 2018) and the SNSF Consolidator grant (2016).
Soft, implantable bioelectronic interfaces for translational research
The introduction of soft materials and microtechnology provides an opportunity to tailor the design of neural implants and explore interfaces with increased selectivity and improved biointegration. Microfabrication allows for customized electrode layouts with micrometric to millimetric electrode diameter, low to high (0.1 to >10 /mm2) electrode density and small to large (mm2 to 10s cm2) surface area. Soft carrier materials and elasticity engineering support neural implants with mechanical signature closer to that of the neural host tissue and potential for long-term biointegration. Multimodal characterization methods are being developed to assess the stability and reliability in vitro of the soft implants under electrochemical and mechanical ageing and envision in vivo validation.
This talk will report on our methodical approach to design, manufacture and test soft neural implants deployed in the central or peripheral nervous systems, and illustrate these concepts with designs from lab-tailored to neuroprosthetic applications.
When: Friday, June 3 @ 11:50 am
Helen S. Mayberg, MD, is a neurologist renowned for her study of brain circuits in depression and for her pioneering deep brain stimulation research, which has been heralded as one of the first hypothesis-driven treatment strategies for a major mental illness. She is the founding Director of Mount Sinai Health System’s The Nash Family Center for Advanced Circuit Therapeutics, a center which advances precision surgical treatments for neuropsychiatric disorders through the rapid conversion of neuroscience and neuroengineering innovations that correct brain circuit abnormalities to restore mood as well as motor and cognitive functioning.
As a behavioral neurologist, Dr. Mayberg has established an international reputation for her pioneering research to map the brain circuits implicated in depression. Early in her career, she developed one of the first “network” models for mood disorders—incorporating fundamentals of neuroanatomy and brain connections with imaging technologies—to propose an alternative neurological view of this classical psychiatric condition that extended beyond the neurochemical models that had dominated for decades. This circuit approach has evolved over the years and continues to anchor many contemporary studies of mood disorders, including the development and latest refinements of deep brain stimulation, a procedure for treatment-resistant depression that involves placing electrodes deep in the brain and turning them on at an amplitude and frequency that disrupts the activity between various brain regions.
Machine Learning Strategies to Track Brain and Behavioral State Changes during DBS Treatment for Depression
Deep Brain Stimulation (DBS) is an experimental treatment strategy for patients with intractable depression. Ongoing research continues to make steady progress, with implementation of refined techniques for surgical targeting and emerging imaging clues as to which patients are most likely to benefit. Close clinical monitoring and systematic long-term follow-up combined with novel rehabilitative strategies has provided additional perspectives on the time course of DBS-mediated effects. The availability of implanted sensing systems now also enables neural monitoring during chronic treatment, providing new tools for treatment optimization and mechanistic perspectives on the trajectory and sustainability of DBS effects. In parallel to ongoing brain recordings and routine clinician and patient self-report ratings, computer vision and machine learning approaches can also be used to detect more subtle changes in core depression-relevant behaviors, developed using high-throughput video analyses of face expression, vocal output and body movements. Examples of hypothesis-driven and model-free analyses using these multimodal datasets will be presented to facilitate discussion of their potential utility to reliably link first-person experiences to quantitative changes in brain state and naturalistic behaviors with depression recovery.
When: Friday, June 3 @ 10:15 am
Serge PICAUD (INSERM Research Director) is Department Chair at the Vision Institute, Paris, France. He has published more than 100 scientific papers on retinal function in normal and pathological conditions. After characterizing the physiology of photoreceptors, he investigated the mechanisms of their degeneration in cell and animal models to develop therapeutic strategies. Recently, he has examined how blue light affects retinal cell survival using an innovative light exposure device. In parallel, he is developing retinal prostheses and microbial opsins to restore vision in blind patients. Clinical translation of these therapeutic approaches has contributed to spinoff companies.
From prostheses to genetic-based brain machine interface for visual restoration
Visual restoration is certainly the greatest challenge for brain-machine interfaces with the high pixel number and high refreshing rate. In the recent year, we brought retinal prostheses and optogenetic therapy up to successful clinical trials. Concerning visual restoration at the cortical level, prostheses have shown efficacy for limited periods of time and limited pixel numbers. We are investigating the potential of sonogenetics to develop a non-contact brain machine interface allowing long-lasting activation of the visual cortex. The presentation will introduce our genetic-based brain machine interfaces for visual restoration at the retinal and cortical levels.
When: Saturday, June 4 @ 9:00 am
Nick Ramsey has a degree in Psychology and a PhD in neuro-psychopharmacology, both from the university of Utrecht (Netherlands). He became a specialist in cognitive neuroimaging in the US (National Institutes of Health), and applies modern techniques, including high-field (7 Tesla) fMRI and intracranial EEG, to questions on working memory, language, and sensorimotor function. His primary goal is to acquire and translate neuro-scientific insights to patients with neurological and psychiatric disorders, with a focus on brain-computer interfacing (BCI). He is full professor in cognitive neuroscience at the department of neurology and neurosurgery of the UMC Utrecht since 2007. He has been awarded several personal grants including a VIDI (NWO, 2002) for elucidating working memory, a VICI (NWO, 2006) and later a European ERC Advanced grant for developing intracranial BCI concepts for paralyzed people. The BCI research resulted in a world-wide first fully implanted BCI for home use, implanted in a locked-in patient with ALS in 2015. Current research aims to develop and implement the next generation BCI implants, for enabling people with communication disorders to speak again. He has co-founded, and is the current President of, the international brain-Computer Interface. He has also been awarded a STW Valorisation grant (phase 1 & 2) for starting a spin-off company (BrainCarta) to provide Clinical fMRI reports to clinicians. He has supervised over 20 PhD students and has (co)authored over 180 peer-reviewed publications.Your content goes here. Edit or remove this text inline or in the module Content settings. You can also style every aspect of this content in the module Design settings and even apply custom CSS to this text in the module Advanced settings.
Intracranial electrodes on the cortical surface: present and future application for Brain-Computer Interfaces to restore communication in people with Locked-in Syndrome
In recent years, several people with complete paralysis have been implanted with Brain-Computer Interface systems to restore communication. In some cases the system requires experts to operate the system, with the goal to develop decoding algorithms. in other cases, the goal was to enable the user to operate the system at home without the help of expert. I will present the current state of affairs in implantable BCI technology intended to restore communication, the avenues for further improvement of system performance, the current hurdles standing in the way of progress, user needs and ethical issues raised by the possibilities offered by implantable BCI systems.
When: Thursday, June 2 @ 11:35 am
Dr. Andrew Schwartz is a Professor of Neurobiology at the University of Pittsburgh. Dr. Schwartz has adjunct appointments at the Center for Neural Basis Cognition (a joint venture of the University of Pittsburgh and Carnegie Mellon University), at the Robotics Institute at Carnegie Mellon University, and at Pitt’s Department of Bioengineering and its Department of Physical Medicine and Rehabilitation.
Dr. Schwartz is a member of the Society for Neuroscience, the Institute of Physics, and the International Psychophysical Society. He has received numerous awards for his pioneering research efforts. Most recently he received the Carnegie Science Award for Life Sciences (2010), the International Brain Mapping & Intraoperative Surgical Planning Society Pioneer in Medicine Award (2010), the Popular Mechanics Breakthrough Award (2012), and the Clinical Research Forum Research Achievement Award (2013).
A motor control perspective
Movement direction is strongly encoded in the firing rate of neurons throughout the nervous system. This robust signaling, recorded from the motor cortex, can be used directly to control prosthetic devices for those suffering from paralysis. In addition, this signaling can be used as a foundation for analytical techniques used to describe the way information propagates through biological networks.
In our applied studies, we have shown how firing rates recorded in parallel from populations of motor cortical neurons can be decoded to control a prosthetic arm and hand with 10 degrees-of-freedom. Using this technology, paralyzed human subjects can operate the prosthetic device to perform sophisticated, natural movements allowing them to carry out a variety of daily-living tasks.
Recently, dynamical system theory has been applied to motor cortical neuronal activity. Of particular interest is the idea that current activity in the motor cortex generates future structured patterns. A simple instantiation of this concept is that of oscillators that are specified before movement begins and then ‘released’ to generate muscle contraction and arm movement. In its basic, form this theory dictates that control takes place autonomously, in the absence of ongoing input.
We are exploring this concept with basic research using behaving monkeys. Our results show how direction signaling drives correlational structure in neuronal populations. Using dimensionality reduction, this structure can be decomposed into independent components. During reaching, the primary components occur sequentially in a way that is linked to features of the behavior. Matching the results of dynamical systems advocates, phase plots of these components show curved trajectories. However instead of interpreting these ‘rotational dynamics’, as a signature of underlying oscillation, we show they are likely due to correlation induced by directional signaling. These results are consistent with the concept of directional signaling acting as a latent driver to neuronal populations.
When: Saturday, June 4 @ 11:50 am
Dr. Shadlen is a professor of neuroscience at Columbia University’s Mortimer B. Zuckerman Mind Brain Behavior Institute. He seeks to understand the brain’s neural mechanisms responsible for reasoning and decision making, as a window on cognition. Using a combination of electrophysiology, imaging, behavior, and computational methods, Shadlen and his team are working in non-human primates and mice to elucidate the neural mechanisms responsible for such tasks as integrating evidence from diverse sources, assigning weight, calculating costs and benefits of anticipated outcomes, processing time, and implementing rules. Shadlen believes that such rudimentary mechanisms are the building blocks of human cognition, and that failures in small sets of these mechanisms and others may be at the root of certain brain disorders.
Neural signals underlying choice and response time on a single decision
Up to now, neural computations leading to choice and response time have been inferred from single neuron responses averaged over many trials. New high-density macaque-neuropixel probes now permit simultaneous recordings from hundreds of neurons in sulci and subcortical structures. I will share our use of these probes to elucidate signals in the lateral intraparietal area (LIP) that underlie formation of perceptual decisions. They allow, for the first time, direct observation of a drift-diffusion signal that gives rise to choice and response time on a single decision. I will contrast the drift-diffusion dynamics in LIP with single-trial dynamics of neurons in the superior colliculus (SC). The burst like dynamics of SC neurons suggest they play a role in terminating the decision process. We confirm this hypothesis by focal inactivation of the SC while recording in area LIP. I will also address a broader issue concerning the analysis of the high-dimensional (multi-neuron) data sets, afforded by high channel recording probes. We find that decoding and factor analyses (e.g., PCA) are capable of discovering important features of the neural computation, but absent hypotheses, they may also serve to obfuscate.
When: Thursday, June 2 @ 2:05 pm
Assistant professor, Department of Neurosciences, KU Leuven, Group leader, NERF and VIB, Member of the Leuven Brain Institute.
My expertise is on R&D of imaging technologies with a focus on functional ultrasound imaging (fUSi) of brain activity. My main objective is to use fUSi in combination with electrophysiological recording and optogenetics/pharmacological control of specific circuits for a better understanding of brain dynamics during multi-sensory stimulation in awake conditions.
At a fundamental level, I’m interested in deciphering the mechanisms for controlling blood supply in normal or pathological conditions where the neurovascular coupling is impaired such as observed in stroke, Alzheimer’s Disease, brain tumors and several pathologies.
10 years of R&D in functional ultrasound imaging (fUSI): What’s next?
Functional ultrasound imaging (fUSI) is based on Doppler ultrasound to track changes in cerebral blood volume as an indirect readout of neuronal activity at high spatiotemporal resolution. fUSI can be performed in head-fixed or freely behaving rodents and allows volumetric images of the entire mouse brain. Proof of concept studies has been conducted in many species, including primates and humans. fUSI has reached maturity, and open access hardware and software solutions developed at NERF contribute to a faster and broader adoption by the neuroscience community. However, it could still be discouraging for nonspecialists to understand the fUSI signal and implement the correct protocol to acquire and analyze the fUSI data. This seminar aims to introduce the physical basis of fUSI and present state-of-the-art in the field while providing detailed examples of the most impactful fUSI research. I will discuss the last capabilities of fUSI for new users and how to avoid potential pitfalls. Finally, I will suggest routes for improving the fUSI technology in the next few years.
When: Friday, June 3 @ 3:05 pm
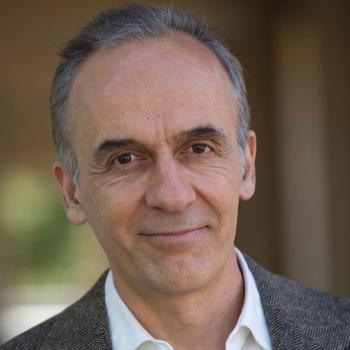
E.J. Chichilnisky
E.J. Chichilnisky is the John R. Adler Professor of Neurosurgery, and Professor of Ophthalmology, at Stanford University, where he has worked since 2013. Previously, he worked at the Salk Institute for Biological Studies for 15 years. He received his B.A. in Mathematics from Princeton University, and his M.S. in mathematics and Ph.D. in neuroscience from Stanford University. His research has focused on understanding the spatiotemporal patterns of electrical activity in the retina that convey visual information to the brain, and their origins in retinal circuitry, using large-scale multi-electrode recordings. His ongoing work now focuses on using basic science knowledge along with electrical stimulation to develop a novel high-fidelity artificial retina for treating incurable blindness.
Toward a High-fidelity Artificial Retina
Electronic interfaces to the retina represent an exciting development in science, engineering, and medicine – an opportunity to exploit our knowledge of neural circuitry and function to restore or even enhance vision. However, although existing devices demonstrate proof of principle in treating blindness, they produce limited visual function. Some of the reasons for this can be understood based on the precise and specific neural circuitry that mediates visual signaling in the retina. Consideration of this circuitry suggests that future devices may need to operate at single-cell, single-spike resolution in order to subserve naturalistic visual function. I will show large-scale multi-electrode recording and stimulation data from the macaque and human retina indicating that, in some cases, such resolution is possible. I will also discuss cases in which it fails, and propose that we can improve artificial vision in such conditions by incorporating our knowledge of the visual system in bi-directional devices that adapt to the host neural circuitry. Finally, I will introduce the Stanford Artificial Retina Project, aimed at developing a retinal implant that more faithfully reproduces the neural code of the retina, and briefly discuss the implications for scientific investigation and for other neural interfaces of the future.
When: Saturday June 4 @ 10:15 am
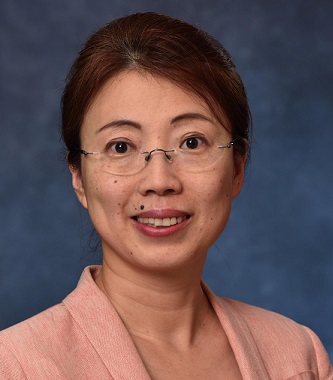
Tracy Cui
Tracy Cui is professor of bioengineering in the Swanson School of Engineering in Pennsylvania. She runs the Neural Tissue/Electrode Interface and Neural Tissue Engineering Lab, where they develop new engineering tools to study and clinically control the interface between tissue and implanted neural devices.
Biomaterials Strategies Towards Seamless Neural Tissue-Device Interface
Neuroelectronic devices placed in the nervous system to record and modulate neuroactivity or monitor neurochemical concentrations present tremendous potentials for understanding neural circuits and treating neurological diseases. Currently, the performance of these devices is sub-optimum due to electrode material limitations and undesired host tissue responses. Quantitative histology and 2-photon imaging have revealed neuronal damage and degeneration, inflammatory gliosis, blood brain barrier leakage and oxidative stress at the site of implants which may compromise the intended recording/stimulation/sensing functions. We use several biomaterial strategies to minimize these responses in order to achieve seamless and stable device-tissue interface. First, conducting polymer-based nanocomposites have been investigated as electrode coatings to facilitate the signal transduction/charge transfer between the ionically conductive tissue and the electronic device. We employ nanotechnology to improve the stability, charge injection and drug delivery capability of the conducting polymers to meet the material challenges. Secondly, materials and devices that mimic the mechanical properties of the neural tissue have been developed and shown to significantly reduce the chronic inflammation. Thirdly bioactive approaches are being developed to modulate the cellular responses for seamless integration. Biomimetic surface modifications have been found to significantly improve neuronal health and inhibit the inflammatory tissue response around the implants. Alternatively, therapeutics that control inflammation, neurodegeneration and oxidative stress can be delivered systemically or locally. These bioactive approaches demonstrated significant effects in improving neural interface performance. The ultimate solution to a seamless device/tissue interface may be a combinatorial approach that takes advantage of multiple biomaterial strategies discussed above and beyond.
When: Saturday, June 4 @ 3:05 pm
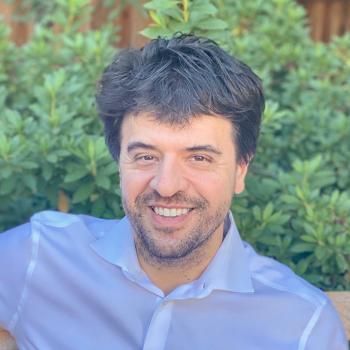
Karl Deisseroth
arl Deisseroth is the D.H. Chen Professor of Bioengineering and of Psychiatry and Behavioral Sciences at Stanford University, and Investigator of the Howard Hughes Medical Institute. He received his undergraduate degree from Harvard, his PhD from Stanford, and his MD from Stanford. He also completed postdoctoral training, medical internship, and adult psychiatry residency at Stanford, and he is board-certified by the American Board of Psychiatry and Neurology. He continues as a practicing psychiatrist at Stanford with specialization in affective disorders and autism-spectrum disease, employing medications along with neural stimulation.
The inner workings of brains and channelrhodopsins
I will discuss the development and application of new optogenetic methods, including molecular tools and brainwide recording approaches (both optical and electrical) in mouse and human; insights into complex behavior that result include a deeper understanding of the mysterious state of dissociation, with both basic-science and clinical implications for the most high-level and integrative roles of the mammalian brain.
I will also explore the remarkable family of light-activated ion channels, or channelrhodopsins, which span three main groups (the cation channels, the anion channels, and the pump-like channels). We have previously described the first high-resolution structures of the first two groups (cation and anion channels), and used these insights to redesign the channels for new kinds of function in optogenetics, but the third family of channelrhodopsins had remained mysterious. For example, ChRmine (the paradigmatic member of this pump-like channelrhodopsin family, for which we reported initial discovery in 2019) exhibits puzzling properties (unusually-large photocurrents, exceptional red-shift in action spectrum, and extreme light-sensitivity) that opened up new opportunities in optogenetics, including the initial definition and control of specific mammalian perceptions at single cell resolution. In this talk I will present our new cryo-electron microscopy structure of ChRmine at 2.0 Å resolution. The structure reveals striking architectural features never seen before in channelrhodopsins including trimeric assembly, a short transmembrane-helix 3 unwound in the middle of the membrane, a prominently-twisting extracellular-loop 1, remarkably-large intracellular cavities and extracellular vestibule, and an unprecedented hydrophilic pore that extends through the center of the trimer, separate from the three individual monomer pores. These structurally distinct features that may enable ChRmine (representing the third of the three major branches of the channelrhodopsin family) to function as an especially potent ion channel; we therefore applied the high-resolution structure to design and create two new proteins (rsChRmine and hsChRmine, for red-shifted and high-speed optogenetic performance, respectively).
Insights into complex behavior that have emerged from Ångstrom-level understanding of these proteins include deeper understanding of the fundamental survival drives of animals at single-cell resolution, and of the highest-level integrative functions of the brain, with both basic-science and clinical implications.
When: Thursday, June 2 @ 4:00 pm
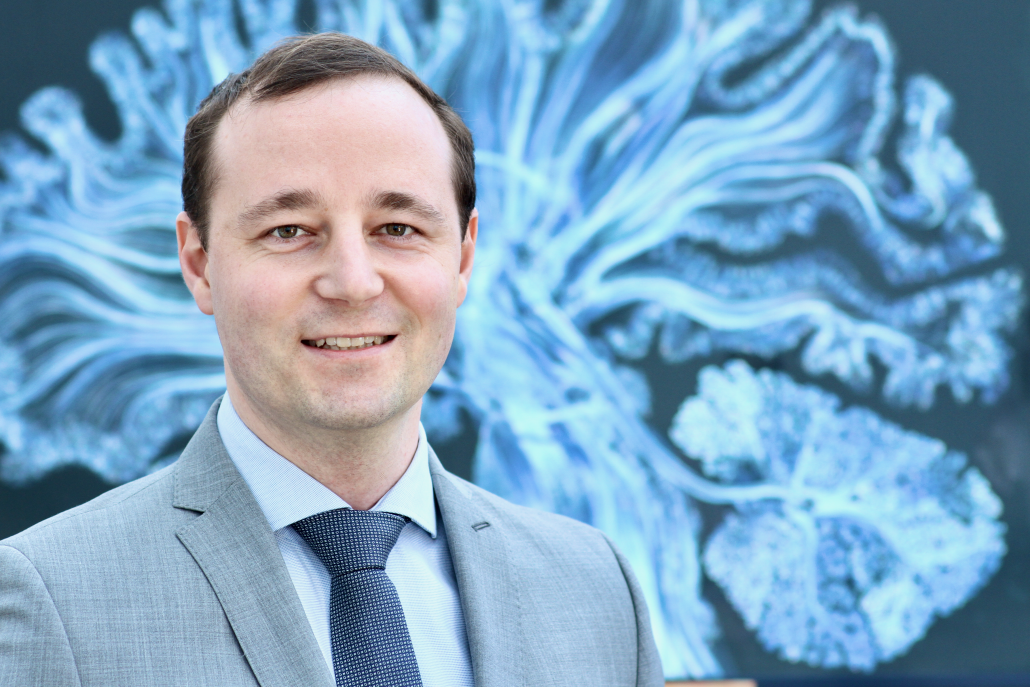
Andreas Horn
Connectomic Deep Brain Stimulation
In recent years, large initiatives around the globe have accumulated data used to calculate average wiring diagrams of the human brain. These connectomes may also be used to investigate clinical populations and have specific value for deep brain stimulation, a procedure in which focal areas in the depth of the brain are being modulated by electrodes invasively implanted into the brains of patients.
These focal stimulations in turn lead to global network effects, often altering activity in distributed whole-brain functional brain networks. Using the human connectome, we are now poised to investigate exactly which networks seem to matter in order to achieve maximal benefits for each patient.
In this talk, I will review methods and results from multiple studies that research labs world wide have applied to study connectomic effects of focal neuromodulation. We will cover results in diseases ranging from the movement disorders spectrum (Parkinson’s Disease, Dystonia, Essential Tremor) to neuropsychiatric (Tourette’s Disease) and psychiatric (Obsessive Compulsive Disorder, Depression) diseases. I will also demonstrate how findings in seemingly different diseases (such as Parkinson’s Disease and Depression) could be transferred to cross-inform one another.
When: Friday, June 3 @ 9:00 am
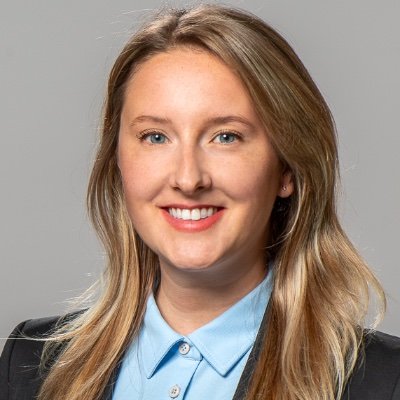
Kristen Kozielski
Magnetoelectric nanomaterials for wireless neuronal modulation
Prof. Kristen Kozielski works with new materials for wireless communication with the brain and nervous system. Her research focuses on understanding and optimizing materials for controlled, electronic signaling to and from the brain. The goal of this work is to contribute to neurotechnologies that are minimally invasive, and possibly implanted with no surgical intervention. Her multidisciplinary group works in materials science, biomaterials, nanotechnology, electrical engineering, and neurobiology.
Kristen completed her PhD in Biomedical Engineering at Johns Hopkins University, and her postdoctoral research at the Max Planck Institute for Intelligent Systems. She moved to the Karlsruhe Institute of Technology in 2019 as a group leader. She has been at the Technical University of Munich (TUM) since 2021, where she is an Assistant Professor of Neuroengineering Materials in the Department of Electrical and Computer Engineering. At TUM, she is also the Program Director of the Elite Master’s Program in Neuroengineering.
When: Saturday, June 4 @ 4:40 pm
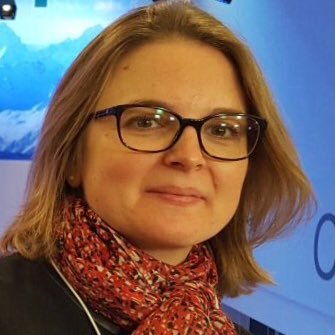
Stéphanie Lacour
She is the recipient of the 2006 MIT TR35, the 2011 Zonta award, and she was selected as one of the 2015 WEF Young Global Leaders. She was awarded the ERC Starting Grant (2011), ERC POC Grants (2016 & 2018) and the SNSF Consolidator grant (2016).
Soft, implantable bioelectronic interfaces for translational research
The introduction of soft materials and microtechnology provides an opportunity to tailor the design of neural implants and explore interfaces with increased selectivity and improved biointegration. Microfabrication allows for customized electrode layouts with micrometric to millimetric electrode diameter, low to high (0.1 to >10 /mm2) electrode density and small to large (mm2 to 10s cm2) surface area. Soft carrier materials and elasticity engineering support neural implants with mechanical signature closer to that of the neural host tissue and potential for long-term biointegration. Multimodal characterization methods are being developed to assess the stability and reliability in vitro of the soft implants under electrochemical and mechanical ageing and envision in vivo validation.
This talk will report on our methodical approach to design, manufacture and test soft neural implants deployed in the central or peripheral nervous systems, and illustrate these concepts with designs from lab-tailored to neuroprosthetic applications.
When: Friday, June 3 @ 11:50 am
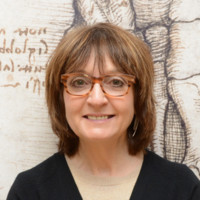
Helen Mayberg
Machine Learning Strategies to Track Brain and Behavioral State Changes during DBS Treatment for Depression
Deep Brain Stimulation (DBS) is an experimental treatment strategy for patients with intractable depression. Ongoing research continues to make steady progress, with implementation of refined techniques for surgical targeting and emerging imaging clues as to which patients are most likely to benefit. Close clinical monitoring and systematic long-term follow-up combined with novel rehabilitative strategies has provided additional perspectives on the time course of DBS-mediated effects. The availability of implanted sensing systems now also enables neural monitoring during chronic treatment, providing new tools for treatment optimization and mechanistic perspectives on the trajectory and sustainability of DBS effects. In parallel to ongoing brain recordings and routine clinician and patient self-report ratings, computer vision and machine learning approaches can also be used to detect more subtle changes in core depression-relevant behaviors, developed using high-throughput video analyses of face expression, vocal output and body movements. Examples of hypothesis-driven and model-free analyses using these multimodal datasets will be presented to facilitate discussion of their potential utility to reliably link first-person experiences to quantitative changes in brain state and naturalistic behaviors with depression recovery.
When: Friday, June 3 @ 10:15 am
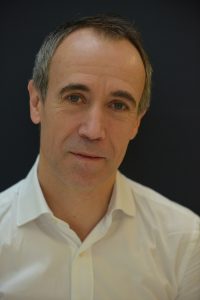
Serge Picaud
From prostheses to genetic-based brain machine interface for visual restoration
Visual restoration is certainly the greatest challenge for brain-machine interfaces with the high pixel number and high refreshing rate. In the recent year, we brought retinal prostheses and optogenetic therapy up to successful clinical trials. Concerning visual restoration at the cortical level, prostheses have shown efficacy for limited periods of time and limited pixel numbers. We are investigating the potential of sonogenetics to develop a non-contact brain machine interface allowing long-lasting activation of the visual cortex. The presentation will introduce our genetic-based brain machine interfaces for visual restoration at the retinal and cortical levels.
When: Saturday, June 4 @ 9:00 am
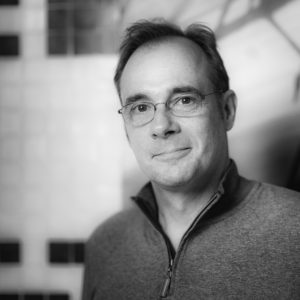
Nick Ramsey
Intracranial electrodes on the cortical surface: present and future application for Brain-Computer Interfaces to restore communication in people with Locked-in Syndrome
In recent years, several people with complete paralysis have been implanted with Brain-Computer Interface systems to restore communication. In some cases the system requires experts to operate the system, with the goal to develop decoding algorithms. in other cases, the goal was to enable the user to operate the system at home without the help of expert. I will present the current state of affairs in implantable BCI technology intended to restore communication, the avenues for further improvement of system performance, the current hurdles standing in the way of progress, user needs and ethical issues raised by the possibilities offered by implantable BCI systems.
When: Thursday, June 2 @ 11:35 am
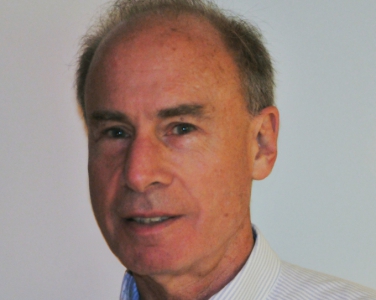
Andy Schwartz
Dr. Schwartz is a member of the Society for Neuroscience, the Institute of Physics, and the International Psychophysical Society. He has received numerous awards for his pioneering research efforts. Most recently he received the Carnegie Science Award for Life Sciences (2010), the International Brain Mapping & Intraoperative Surgical Planning Society Pioneer in Medicine Award (2010), the Popular Mechanics Breakthrough Award (2012), and the Clinical Research Forum Research Achievement Award (2013).
A motor control perspective
Movement direction is strongly encoded in the firing rate of neurons throughout the nervous system. This robust signaling, recorded from the motor cortex, can be used directly to control prosthetic devices for those suffering from paralysis. In addition, this signaling can be used as a foundation for analytical techniques used to describe the way information propagates through biological networks.
In our applied studies, we have shown how firing rates recorded in parallel from populations of motor cortical neurons can be decoded to control a prosthetic arm and hand with 10 degrees-of-freedom. Using this technology, paralyzed human subjects can operate the prosthetic device to perform sophisticated, natural movements allowing them to carry out a variety of daily-living tasks.
Recently, dynamical system theory has been applied to motor cortical neuronal activity. Of particular interest is the idea that current activity in the motor cortex generates future structured patterns. A simple instantiation of this concept is that of oscillators that are specified before movement begins and then ‘released’ to generate muscle contraction and arm movement. In its basic, form this theory dictates that control takes place autonomously, in the absence of ongoing input.
We are exploring this concept with basic research using behaving monkeys. Our results show how direction signaling drives correlational structure in neuronal populations. Using dimensionality reduction, this structure can be decomposed into independent components. During reaching, the primary components occur sequentially in a way that is linked to features of the behavior. Matching the results of dynamical systems advocates, phase plots of these components show curved trajectories. However instead of interpreting these ‘rotational dynamics’, as a signature of underlying oscillation, we show they are likely due to correlation induced by directional signaling. These results are consistent with the concept of directional signaling acting as a latent driver to neuronal populations.
When: Saturday, June 4 @ 11:50 am
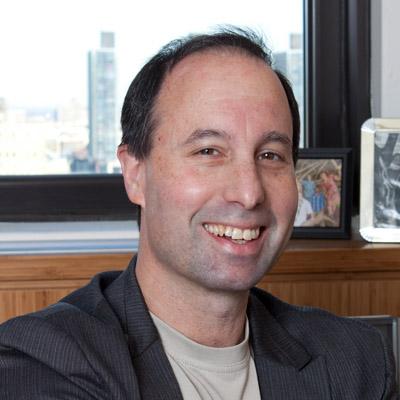
Michael Shadlen
Neural signals underlying choice and response time on a single decision
Up to now, neural computations leading to choice and response time have been inferred from single neuron responses averaged over many trials. New high-density macaque-neuropixel probes now permit simultaneous recordings from hundreds of neurons in sulci and subcortical structures. I will share our use of these probes to elucidate signals in the lateral intraparietal area (LIP) that underlie formation of perceptual decisions. They allow, for the first time, direct observation of a drift-diffusion signal that gives rise to choice and response time on a single decision. I will contrast the drift-diffusion dynamics in LIP with single-trial dynamics of neurons in the superior colliculus (SC). The burst like dynamics of SC neurons suggest they play a role in terminating the decision process. We confirm this hypothesis by focal inactivation of the SC while recording in area LIP. I will also address a broader issue concerning the analysis of the high-dimensional (multi-neuron) data sets, afforded by high channel recording probes. We find that decoding and factor analyses (e.g., PCA) are capable of discovering important features of the neural computation, but absent hypotheses, they may also serve to obfuscate.
When: Thursday, June 2 @ 2:05 pm
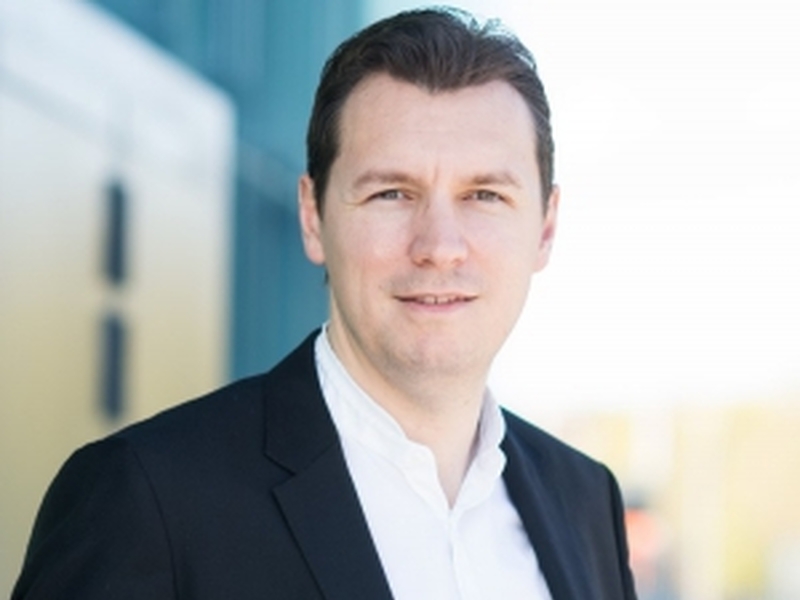
Alan Urban
Assistant professor, Department of Neurosciences, KU Leuven, Group leader, NERF and VIB, Member of the Leuven Brain Institute.
My expertise is on R&D of imaging technologies with a focus on functional ultrasound imaging (fUSi) of brain activity. My main objective is to use fUSi in combination with electrophysiological recording and optogenetics/pharmacological control of specific circuits for a better understanding of brain dynamics during multi-sensory stimulation in awake conditions.
At a fundamental level, I’m interested in deciphering the mechanisms for controlling blood supply in normal or pathological conditions where the neurovascular coupling is impaired such as observed in stroke, Alzheimer’s Disease, brain tumors and several pathologies.
10 years of R&D in functional ultrasound imaging (fUSI): What’s next?
functional ultrasound imaging (fUSI) is based on Doppler ultrasound to track changes in cerebral blood volume as an indirect readout of neuronal activity at high spatiotemporal resolution. fUSI can be performed in head-fixed or freely behaving rodents and allows volumetric images of the entire mouse brain. Proof of concept studies has been conducted in many species, including primates and humans. fUSI has reached maturity, and open access hardware and software solutions developed at NERF contribute to a faster and broader adoption by the neuroscience community. However, it could still be discouraging for nonspecialists to understand the fUSI signal and implement the correct protocol to acquire and analyze the fUSI data. This seminar aims to introduce the physical basis of fUSI and present state-of-the-art in the field while providing detailed examples of the most impactful fUSI research. I will discuss the last capabilities of fUSI for new users and how to avoid potential pitfalls. Finally, I will suggest routes for improving the fUSI technology in the next few years.
When: Friday, June 3 @ 3:05 pm